What is Cell Fractionation?
Despite the fact that biochemical study requires the destruction of the cell’s anatomy, gentle fractionation methods have been devised to separate the different cell components while preserving their functions. With these amazing procedures, you can divide a cell into its working organelles and macromolecules in the same way you can split a tissue into its live constituent cell types.
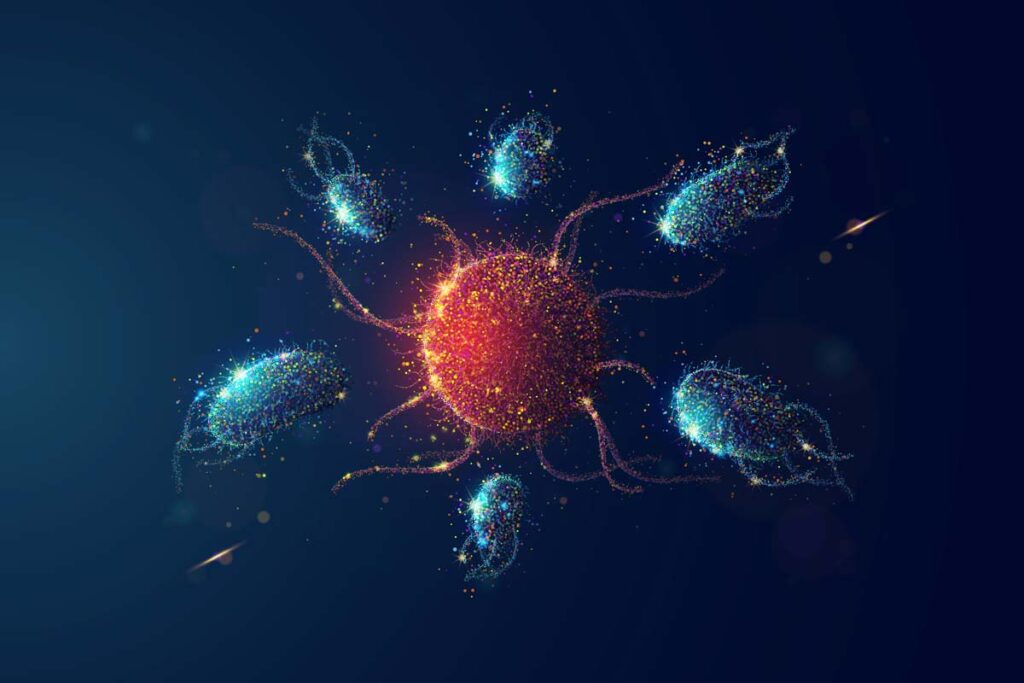
Cell fractionation is a technique for separating cellular components while keeping their distinct functions. This technique initially showed where various biochemical activities occur within the cell.
Subcellular fractionation produces an enriched supply of protein for further purification and aid in detecting certain diseases.
Cell fractionation allows you to study the many components of a cell separately. For example, you can see which organelles produce the most energy after the organelles are isolated.
Let’s take a deeper look into the processes and steps involved in cell fractionation below:
Extraction
Extraction is the first stage in the process of separating any subcellular structures that may exist. To ensure that organelles and biomolecules retain their biological function under mild conditions (known as cell-free systems) before being used, you have to maintain isotopic constraints.
At 0-40°C, the cells or tissues suspend in a solution with an adequate pH and salt content, typically an isotonic solution of sucrose (0.25 mol/L) with a pH of 7.0.
Homogenization/ Breaking The Cells
To get to the information contained within anything, you must first open it. The same is true for cells. You can achieve homogenization through either of the following:
- Grinding
- High pressure
- Osmotic shock
Detergents allow the cell membrane to open up, allowing access to the contents within the cell. Because detergents can interact with both membranes and elements of the cell that are soluble in water, they disrupt cellular membranes.
In addition, detergents allow cellular components to be mixed or homogenized because they interact with both lipid (membrane) and soluble (cytoplasmic) elements of the cell.
A cell homogenate, or cell lysate, is created when the cellular components are mashed together. In truth, detergents and soaps remove oil and grease by allowing substances that do not ordinarily combine with water to dissolve in water and rinsed away.
In most cases, lysing or opening the cells in detergent is supplemented with a physical approach that further breaks the cell, such as blender-like devices, glass beads, or breaking the cell with sound energy.
Physical methods combined with detergents ensure that all cells in the sample break, allowing you to isolate as much of your cellular fraction as feasible. Modern Biology Inc. can provide you with experiment kits and lesson plans to put these concepts into practice.
Separation
Centrifugation is a technique for separating cell homogenates into fractions by spinning them at high speeds for an extended period. If you have ever been on an anti-gravity carnival ride, you will better understand how centrifugation works. These rides gradually accelerate to the point that you are forced against the walls and no longer require the use of a seat belt to stay upright.
In a similar vein, centrifugation generates forces that are hundreds of times greater than those produced by gravity, forcing cellular components to the bottom of the container in which they are.
A preparative ultracentrifuge separates (fractionate) the various components of the homogenate through a series of centrifugation. A metal rotor with cylindrical holes to accommodate centrifuge tubes and a motor that spins the rotor fast enough to generate centrifugal forces make up the ultracentrifuge. Theodor Svedberg (1926) invented the ultracentrifuge, which he used to calculate hemoglobin’s molecular weight.
Even small molecules like t-RNA and enzymes can sediment and separate from other components in today’s ultracentrifuges, which rotate at speeds up to 80,000 rpm (rpm=rotations per minute) and exert a gravitational pull of around 500,000 g. It is paramount to keep the ultracentrifuge chamber at a high vacuum to eliminate friction, prevent heating, and keep the sample at 0-4°C.
The determinant of the rate at which each component settles down during centrifugation is by its size and shape and is expressed in terms of the sedimentation coefficient, also known as the Svedberg unit or S-value, where IS = 1 x 10-13 seconds.
Modern Day application of cell Fractionation
From the above information, you have learned what cell fractionation is and how to perform the experiments. However, you need to know how this particular science affects our lives and whether or not it adds value to our general well-being. So, what are some applications of cell fractionation?
Decipher the Molecular Details of Complex Cellular Processes.
The ultracentrifuge has greatly aided our understanding of the functions of many cellular components by isolating organelles and other significant subcellular components.
Experiments on centrifuge-purified mitochondria and chloroplasts, for example, revealed the fundamental role of these organelles in transforming energy into forms that the cell can use. Similarly, resealed vesicles (microsomes) produced from rough and smooth endoplasmic reticulum fragments have been isolated and examined as functional models of these compartments of the complete cell.
Using purified cell-free systems, scientists can extend this approach to examine many different biological processes free of all complicated side reactions in a living cell. They fractionate cell homogenates in this scenario to cleanse each of the constituents of macromolecules required to catalyze a biological process.
For example, experiments that focused on a cell homogenate that enabled RNA translation to create proteins were used to learn the mechanics of protein synthesis.
Step by step, fractionation of this homogenate produced ribosomes, tRNAs, and other enzymes, which collectively make up the protein-synthetic machinery.
You could add individual pure components or withhold one at a time to determine their particular role in the overall process. The restoration of every biological activity in a purified cell-free environment to characterize all its components and action mechanisms is crucial today.
Protein Fractionation
The most common method for fractionating proteins is column chromatography, which involves passing a protein solution down the column containing a porous solid matrix. The interaction of the different proteins with the matrix slows them down to varying degrees, and they can be collected as they flow out of the bottom of the column.
Proteins can be separated based on their charge (ion-exchange chromatography), hydrophobicity (hydrophobic chromatography), size (gel-filtration chromatography), or ability to bind to specific small molecules or other macromolecules, depending on the matrix used (affinity chromatography).
Commercially accessible matrices come in a variety of shapes and sizes. Proteins separate according to the distribution of charges on their surface using ion-exchange columns, packed with tiny beads with either a positive or negative charge. Proteins with exposed hydrophobic regions are slow in hydrophobic columns filled with beads from which hydrophobic side chains protrude.
Gel-filtration columns, which separate proteins based on their size, are with tiny, porous beads: molecules small enough to pass through the pores linger inside successive dots as they pass down the column. Larger molecules remain in the solution flowing between the beads and thus move more quickly, emerging first from the column.
Therefore, Gel-filtration chromatography is a convenient approach to determine the size of molecules and separating them.
You can perform biochemical analysis of cell populations by disrupting them and fractionating their contents using ultracentrifugation. Further fractionations enable functional cell-free systems, which are necessary for determining the molecular intricacies of complicated biological processes. You can use this method to investigate protein synthesis,
DNA replication, RNA splicing, the cell cycle, mitosis, and many types of intracellular transport. SDS polyacrylamide-gel electrophoresis can detect the molecular weight and subunit composition of even tiny amounts of a protein.
Proteins are resolved as discrete spots in two-dimensional gel electrophoresis by isoelectric focusing in one dimension, followed by SDS polyacrylamide-gel electrophoresis in the other. Thus, even proteins that are ordinarily insoluble in water can be separated using electrophoresis.
Column chromatography can purify the main proteins insoluble cell extracts; physiologically active proteins can be separated based on their molecular weight, hydrophobicity, charge properties, or affinity for other molecules, depending on the type of column matrix.
In a typical purification, the sample is passed through a series of columns in order, with the enriched fractions from one column applied to the next. Thus, scientists can now study the biological functions of a protein in-depth once it is purified. In addition, you can quickly determine the masses of proteins and peptides produced from them, causing mass spectrometry. With this knowledge, may use genome databases to deduce the protein’s remaining amino acid sequence from the nucleotide sequence of its gene.
Learn More About Cell Fractionation
Much of what we know about cellular, molecular biology has come from researching cell-free systems. Such studies figure out the molecular aspects of DNA replication and transcription, RNA splicing, protein translation, muscle contraction, and particle transport through microtubules, to name a few examples.
Cell-free systems are used to study the cell division cycle, chromosome separation on the mitotic spindle, and the vesicular-transport processes involved in the transfer of proteins from the endoplasmic reticulum via the Golgi apparatus to the plasma membrane. In theory, cell homogenates also serve as the beginning material for completely separating all the cell’s separate macromolecular components.